Deoxy[Hb+Mb] increases consistently up to peak oxygen consumption in elite cyclists
International Journal of Applied Sports Sciences, Vol.30, No.1, pp.11-19
https://doi.org/10.24985/ijass.2018.30.1.11
초록
This study aimed to compare deoxygenation in the vastus lateralis in the skeletal muscle microvasculature of nine world-class/elite (WC/E) vs. thirty-one well-trained/trained (T) cyclists by near infrared spectroscopy (NIRS) during a maximal aerobic power (MAP) test. Interaction analysis showed that 1st degree polynomial trends across % peak oxygen consumption (%VO2peak) differed significantly between WC/E and T cyclists on deoxygenated hemoglobin-myoglobin (Δ[HHb]) and on total hemoglobin-myoglobin (Δ[THb]) from 70 to 100% of VO2peak. These results show that the linear slopes of these trends were different. Near-plateau of Δ[HHb] was observed in T cyclists, whereas a consistent increase up to VO2peak was seen in WC/E cyclists. The Δ[THb] curve declined in T cyclists suggesting that blood volume in the vastus lateralis microcirculation decreases from 70 to 100% of VO2peak whereas it increases slowly but continuously to 95% of VO2peak in WC/E cyclists. The results of this study showed that deoxygenation in the vastus lateralis increases consistently up to VO2peak in WC/E cyclists and seems to be influenced by blood volume in the microcirculation.
Abstract
This study aimed to compare deoxygenation in the vastus lateralis in the skeletal muscle microvasculature of nine world-class/elite (WC/E) vs. thirty-one well-trained/trained (T) cyclists by near infrared spectroscopy (NIRS) during a maximal aerobic power (MAP) test. Interaction analysis showed that 1st degree polynomial trends across % peak oxygen consumption (%VO2peak) differed significantly between WC/E and T cyclists on deoxygenated hemoglobin-myoglobin (Δ[HHb]) and on total hemoglobin-myoglobin (Δ[THb]) from 70 to 100% of VO2peak. These results show that the linear slopes of these trends were different. Near-plateau of Δ[HHb] was observed in T cyclists, whereas a consistent increase up to VO2peak was seen in WC/E cyclists. The Δ[THb] curve declined in T cyclists suggesting that blood volume in the vastus lateralis microcirculation decreases from 70 to 100% of VO2peak whereas it increases slowly but continuously to 95% of VO2peak in WC/E cyclists. The results of this study showed that deoxygenation in the vastus lateralis increases consistently up to VO2peak in WC/E cyclists and seems to be influenced by blood volume in the microcirculation.
Introduction
The balance between O2 supply and O2 demand is a major determinant of exercise capacity. Studying deoxygenation in the skeletal muscle microvasculature during exercise, where gas exchange occurs, could be important to unearth potential physiological limitations to endurance performance. Near infrared spectroscopy (NIRS) has been shown to be a suitable, non-invasive way of measuring deoxygenation and blood volume in the skeletal muscle microvasculature. It has served to examine the dynamics of local oxygen delivery and consumption within the skeletal muscle microvasculature. We noted that an increasing number of coaches and athletes are using the NIRS in cycling.
Concentration changes of the NIRS-derived deoxygenated hemoglobin-myoglobin (Δ[HHb]) signal have been measured in several studies to assess changes in deoxygenation during incremental ramp exercises up to exhaustion (Boone, Koppo, Barstow, & Bouckaert, 2009; Boone, Koppo, Barstow, & Bouckaert, 2010; Ferreira, Koga, & Barstow, 2007; McNarry, 2010; Murias, Keir, Spencer, & Paterson, 2013a; Murias, Spencer, Keir, & Paterson, 2013b; Spencer, Murias, & Paterson, 2012). These investigations have demonstrated that the Δ[HHb] pattern measured in the vastus lateralis microvasculature presents a sigmoidal profile during an incremental ramp exercise up to maximal oxygen consumption (VO2max). This sigmoidal profile has been challenged (Spencer et al., 2012), and an alternative “double-linear” model has been proposed. The second component of this model has been reported to “level out”, indicating a Δ[HHb]-break point (BP). In the sigmoidal profile, the above-mentioned authors also observed an approximately linear “plateau” at high intensities during incremental ramp exercises. The studied participants were highly-trained cyclists (peak oxygen consumption (VO2peak) ≈ 65 mlO2·kg-1·min-1) (Boone et al., 2009; Boone et al., 2010), young, healthy, active men and women (≈ 48-52 mlO2·kg-1·min-1) (Boone et al., 2009; Ferreira et al., 2004; Murias et al., 2013a; Spencer et al., 2012), and trained and untrained pre-, peri- and postpubertal girls (McNarry, 2010). However, to our knowledge, the development pattern of Δ[HHb] has not been measured in world-class/elite (WC/E) cyclists during an incremental exercise test to exhaustion.
It is, therefore, particularly interesting to study skeletal muscle deoxygenation in WC/E cyclists to understand the impact of many years of aerobic training and competing at national and international levels. Boone et al. (2009) and McNarry (2010) demonstrated that the pattern of Δ[HHb] in vastus lateralis was influenced by aerobic fitness status during an incremental test, but WC/E cyclists were not included in their experiments.
The aim of the present study was to compare the dynamics of deoxygenation in the vastus lateralis during a maximal aerobic power (MAP) test between WC/E and well-trained/trained (T) cyclists.
Materials and methods
Study participants
Forty healthy male cyclists participated in this study, including 17 road cyclists, 16 cross-country mountain bikers and 7 triathletes. Before presenting to the laboratory, they were asked not to practice any intense physical activity during the 48 h preceding each test. They were also asked to refrain from consuming tobacco and caffeine at least 2 h before the tests, and from alcohol and drugs for at least 24 h. All study participants had performed a MAP test previously in their training background. The study was approved by the UQTR Research Ethics Committee, and written informed consent was obtained from each participant after an explanation of all study tests and procedures.
Experimental design
Two groups were formed according to training and race status criteria of Jeukendrup, Craig, and Hawley (2000). As recommended by these authors, E and WC cyclists are ranked by the Union Cycliste Internationale (UCI). In the present study, the first group (WC/E) comprised 9 cyclists with a UCI ranking and classified as world-class/elite, while the second group (T) was composed of 31 cyclists without UCI ranking and deemed well-trained or trained. Each participant completed a MAP test at their laboratory visit. The height and weight (Tanita BF-350 Body Composition Analyzer, Tanita, Tokyo, Japan) of each participant were measured before the test. The test was administered in a laboratory where the ambient temperature was maintained at 21°C.
Maximal aerobic power test
On their visit to the laboratory, participants were tested to measure their VO2peak. Oxygen consumption (VO2) was assessed with a gas analyzer (Moxus, AEI Technologies, Pittsburgh, PA, USA). Each participant wore a Hans Rudolph 2700 series body style saliva trap 2-way NRBV mask (Hans Rudolph, Kansas City, MO, USA) with head support for Rudolph series 2726 valves. The device was calibrated prior to the test, according to the manufacturer’s instructions, with 4 different gases of known concentrations. Flow volume was calibrated with a 3.00-L Hans Rudolph 5530 series calibration syringe. In each case, participants were asked to bring and use their own road bicycles. Thus, all participants had the same type of bicycle. The bicycles were mounted on a Computrainer ergometer (Racermate, Computrainer Lab, Seattle, WA, USA) which had been validated (Cane, Seidman, Sowash, & Otto, 1996). Participants pedaled at their own preferred cadence (90-100 rpm) throughout the test, which began at 130 watts and was stepped up by 30-watts increments until they reached volitional exhaustion and/or pedaling rate could not be maintained over 90 rpm. Duration of the effort periods was 5 min, separated by 3-min recovery periods at 1 watt/kg body weight. VO2peak was defined as the highest VO2 achieved during the test averaged over a 30-s interval. Maximal aerobic power (MAP) was defined as the highest power reached causing a minimal increase of 150 ml of oxygen consumption from the previous power.
Near infrared spectroscopy
Participants also wore a portable NIRS (Portamon, Artinis Medical Systems BV, Utrecht, the Netherlands) during the test. NIRS is a non-invasive method of monitoring local muscle oxy/deoxygenation. Oxyhemoglobin-myoglobin (Δ[HbO2]), deoxyhemoglobin-myoglobin (Δ[HHb]) and total hemoglobin-myoglobin (Δ[HbO2] + Δ[HHb] = Δ[THb]) were measured in the vastus lateralis muscle. These signals were “zeroed” during resting baseline and reported as relative differences in concentration during exercise. The Δ[HHb] signal from the NIRS is less sensitive than Δ[HbO2] to changes in blood volume under the probe and has been considered as an estimate of deoxygenation in the microcirculation (Ferreira, Townsend, Lutjemeier, & Barstow, 2005; Grassi et al., 2003). Therefore, the HHb data were used to investigate dynamic increases in microvascular deoxygenation during MAP testing. The NIRS-derived THb signal is a reliable indicator of blood volume changes in interrogation sites of working muscles (De Blasi et al., 1994).
To record the NIRS signal, the probe was placed over the right vastus lateralis muscle, approximately 10-12 cm from the knee joint, along the vertical axis of the thigh (Belardinelli, Barstow, Porszasz, & Wasserman, 1995). The thigh, with attached optodes and covering, was wrapped with elastic bandage to minimize optode movement while still permitting freedom of cycling movements. Black cloths were wrapped around the probe and the skin to prevent contamination by ambient light. The device was placed in a clear plastic bag to prevent distortion of the signal caused by sweating during exercise. The skin was carefully shaven previously. NIRS signals were collected at rest and during exercise at a sampling frequency of 10 Hz. Baseline measurements were recorded while participants were seated on their own bicycle with their right leg in a relaxed position at the lowest point of the pedal (bottom dead center). A setting time of 120 s was allowed on each test for signal stabilization before baseline (resting value) of 60 s was recorded.
Data analysis
Values reported in Figure 1 were obtained for each group by averaging individual curves, each curve being standardized from 70% to 100% of VO2peak by increments of 5% using 2nd degree linear interpolation. The data considered for interpolation were the last 30-s averages of every 5-min effort period. Δ[HHb], Δ[HbO2], Δ[THb], Δ[HHb]/VO2 and respiratory equivalent for oxygen (VE/VO2) values were examined only from 70 to 100% of VO2peak because, for some cyclists of the T group, the workload of 130 watts (first step of the incremental test) corresponded to VO2 of approximately 70% of their VO2peak.
Figure 1.
*** p ≤ 0.001 indicates significant 1st degree polynomial difference between WC/E and T.
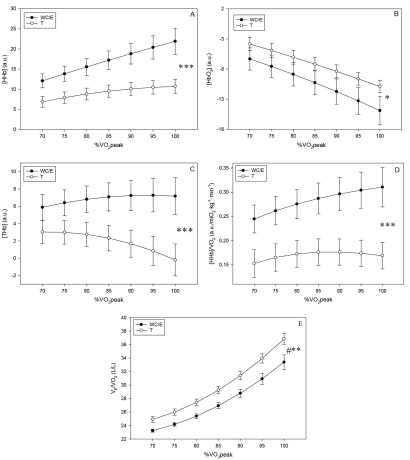
Statistical analysis
Independent t-tests were performed to assess significance between WC/E and T for sets of specific parameter values. Two factor ANOVAs with repeated measures were used to assess global difference between groups. The differential evolution of each variable was tested as an interaction of 1st and 2nd order polynomial trends between WC/E and T. Graphics were created with Sigma Plot 10.0 (San Jose, CA, USA). Statistics were analyzed with IBM SPSS Statistics 20 (Chicago, IL, USA) and Microsoft Excel 2007. Data in Table 1 are presented as means ± SD (standard deviation). Data in Figures 1 and 2 are presented as means ± SEM (standard error of the mean). Calculations were done with the harmonic mean solution for unequal group sizes (Winer, Brown, & Michels, 1991). Statistical significance was set at p ≤ 0.05.
Table 1.
Descriptive data on study participants
Variables | Total group (N=40) | WC/E (N=9) |
T (N=31) |
---|---|---|---|
Age (years) | 30.4 ± 9.3 | 24.3 ± 8.2 | 32.1 ± 8.9* |
Weight (kg) | 72.5 ± 7.5 | 74.8 ± 7.9 | 71.8 ± 6.9 |
Body mass index (kg/m2) | 23.4 ± 2.1 | 22.4 ± 1.9 | 23.6 ± 2.2 |
VO2peak (mlO2·kg-1·min-1) | 63.4 ± 7.8 | 69.4 ± 7.0 | 61.7 ± 7.2** |
MAP (watts) | 316 ± 44 | 380 ± 23 | 297 ± 27*** |
Relative MAP (watts/kg) | 4.4 ± 0.6 | 5.1 ± 0.6 | 4.2 ± 0.5*** |
TTE (s) | 2,189 ± 470 | 2,860 ± 356 | 1,994 ± 270*** |
Results
Table 1 reports descriptive physiological and anthropometric measurements of the 40 study participants and those of WC/E and T.
Interaction analysis (Groups × %VO2peak, see Figure 1) showed that 1st degree polynomial trends differed between WC/E and T on the Δ[HHb] variable (F = 49.90, df = 1 and 228, p ≤ 0.001), on the Δ[HbO2] variable (F = 5.88, df = 1 and 228, p ≤ 0.05), on the Δ[THb] variable (F = 28.87, df = 1 and 228, p ≤ 0.001), on the Δ[HHb]/VO2 variable (F = 13.96, df = 1 and 228, p ≤ 0.001) and on the VE/VO2 variable (F = 7.85, df = 1 and 228, p ≤ 0.01): from 70 to 100% of VO2peak. As for 2nd degree polynomials, no significant variance was attached to them. Whereas Δ[HHb] increased consistently while Δ[THb] plateaued in WC/E, Δ[HHb] tended to plateau in T as Δ[THb] displayed a reverse (decreased) effect.
Moreover, from 70 to 100% of VO2peak, VE/VO2 was lower (F = 5.52, p ≤ 0.05, Figure 1E) in WC/E than in T.
Discussion
To our knowledge, this is the first study to examine Δ[HHb] dynamics in WC/E cyclists during MAP testing. The novelty of the study design resided in assessing the dynamics of these variables in WC/E cyclists. The main findings were that, in contrast to T cyclists, deoxygenation in the vastus lateralis increased consistently in WC/E cyclists during the MAP test from 70 to 100% of VO2peak.
Murias et al. (2013a) and Spencer et al. (2012) observed that the slope of the second component of the %Δ[HHb] double-linear model was approximately zero, indicating an approximately linear plateau in response at the end of the ramp exercise (from ~80% of VO2peak). This suggests that, in young, healthy, active men, the amount of oxygen that can be extracted is limited toward the end of ramp incremental exercises. The present study discerned significant differences between 1st degree polynomial trends in WC/E and T cyclists (Figure 1A), showing that the linear slopes of these trends were different. We also observed that, in T cyclists, Δ[HHb] increased less and less up to VO2peak. In contrast, the dynamics of Δ[HHb] increased consistently up to VO2peak in WC/E cyclists. It implies that there is no discernable limit to the amount of oxygen that can be extracted toward the end of maximal aerobic power tests in these cyclists.
Δ[THb] was assessed in an attempt to elucidate the physiological significance of the deoxygenation near-plateau during incremental exercise in T cyclists. We observed that 1st degree polynomial trends differed significantly between WC/E and T cyclists (Figure 1C), showing that the linear slopes of these trends were different. We noted that, from 70% of VO2peak, the Δ[THb] curve declined in T cyclists. The Δ[THb] dynamic in T cyclists indicates that blood volume in the vastus lateralis microcirculation decreases toward the last third of the test whereas it increases slowly but continuously to 95% of VO2peak in WC/E cyclists. The increase of blood volume could influence the amount of available oxygen in WC/E cyclists in the vastus lateralis during a MAP test. The combination of the near-plateau of Δ[HHb] and the constant decrease of Δ[HbO2] in T suggest that the oxygen extraction plateaued and that the amount of available oxygen decreased, probably explained by a decrease of oxygen supply in microcirculation. Several putative mechanisms have been proposed to explain the different dynamics of blood volume between WC/E and T cyclists. Kjaer (1998) demonstrated that training reduced catecholamine spillover during exercise and induced larger arteriolar dilatation. Mortensen et al. (2014) found that exercise training reduced the vasoconstrictor response to sympathetic nerve activity and improved the ability to override sympathetic vasoconstrictor activity. Some authors observed a positive correlation between skeletal muscle oxidative capacity and β-adrenoreceptor density in endurance-trained rats (Buckenmeyer, Goldfarb, Partilla, Pineyro, & Dax, 1990). Kjaer (1998) reported that endurance-trained athletes had higher epinephrine secretion capacity at given relative intensities – development of the so-called “sports adrenal medulla”. Δ[THb] could also be related to improvement in endothelium-dependent vasodilator capacity which, in turn, is related to aerobic capacity (Kasikcioglu et al., 2005). All these improvements could lead to increased microvasculature vasodilation.
In addition, the higher percentage or recruitment of oxidative slow-twitch fibers may delay the conscription of fast-twitch fibers during sub-maximal intensity in endurance athletes (Costill et al., 1976; Trappe et al., 2006). Slow-twitch fibers are more sensitive to endothelium-dependent vasodilation agents (Wunsch, Muller-Delp, & Delp, 2000; Woodman et al., 2001) and lower sympathetically-mediated vasoconstriction (Folkow & Halicka, 1968; Laughlin & Armstrong, 1987; Delp & Armstrong, 1988). Finally, it has been demonstrated that capillary density is increased with endurance training and is related to blood capacitance of the capillary network (Saltin, 1988) as well as changes in arteriolar diameter and tone (Delp, 1997).
Δ[HHb]/VO2 dynamic was also assessed in an attempt to elucidate the physiological significance of the deoxygenation near-plateau during incremental exercise in T cyclists. We noted that, from 90% of VO2peak, the Δ[HHb]/VO2 curve declined in T cyclists. The Δ[HHb]/VO2 dynamic in T cyclists suggests that deoxygenation in the vastus lateralis microcirculation per mlO2∙kg-1∙min-1 of systemic oxygen consumption decreased toward the end of the test whereas it increased slowly but continuously to VO2peak in WC/E cyclists. Increasing systemic VO2 in the presence of a near-plateau of deoxygenation indicates that oxygen requirements in other areas (e.g., respiratory muscles) surge at high intensities (Harms et al., 1998) while deoxygenation in the vastus lateralis plateaus. The significant difference of VE/VO2 in Figure 1E shows that for each liter of oxygen consumed, more air liters are ventilated by T cyclists from 70 to 100% of VO2peak, probably resulting in a greater work of respiratory muscles and thus higher oxygen requirements. This potential mechanism could also explain the difference of blood volume in the vastus lateralis in the present study between WC/E and T cyclists measured by Δ[THb].
Murias et al. (2013a) observed that Δ[HHb]-BP was associated with respiratory compensation point and proposed a relationship between ventilatory responses to heavier exercise and local oxygen transport/extraction in young, healthy, active men and women. However, this relationship does not seem to exist among WC/E cyclists because, in the present study, we did not see any Δ[HHb]-BP. Further research is needed to elucidate the fact that no Δ[HHb]-BP occurred in WC/E cyclists.
We chose to compare the skeletal muscle deoxygenation of WC/E and T cyclists on an incremental step test. As cited previously, several studies used a ramp exercise to assess the Δ[HHb] pattern up to exhaustion. However, Boone et al. (2010) observed that the Δ[HHb] response follows a similar pattern in incremental step and ramp exercises in a group of trained cyclists. This suggests that results of the present study were in all likelihood not influenced by the exercise protocol.
We also observed a significant difference of age between WC/E and T cyclists (Table 1). However, Gravelle, Murias, Spencer, Paterson, & Kowalchuk (2012) compared two groups of healthy and recreationally men (25 ± 5 vs. 70 ± 3 years) and reported that no age-related differences in the [HHb] pattern were reported when work rate was expressed as a percentage of peak work rate. Thus, it is unlikely that age could influence [HHb] pattern in our study considering that WC/E and T cyclists were only eight years of age apart.
It seemed also pertinent to consider the individual curves of Δ[HHb]. We observed that the mean Δ[HHb] curves in WC/E and T (Figure 1A) don’t necessarily reflect the Δ[HHb] profile of every cyclists of these groups. There were also cyclists in T group whose Δ[HHb] increased consistently up to VO2peak. In fact, in T, Δ[HHb] increased consistently up to VO2peak in 7 cyclists out of 31 (23%) whereas it was the case in 5 cyclists out of 9 (56%) in WC/E (p ≈ 0.035, binomial test). Thus, we noted that a Δ[HHb] near-plateau also occurred in some cyclists in the WC/E. A more detailed analysis about the training history and program of cyclists would be needed to see what principal parameter(s) could have improved the Δ[HHb] profile in high intensities. This seems especially important if coaches start using the plateau analysis as a training tool.
Conclusions
We examined Δ[HHb] dynamics in WC/E cyclists during MAP testing. The results show that the pattern of skeletal muscle deoxygenation in the vastus lateralis microcirculation during MAP testing is different from 70 to 100% of VO2peak between cyclists of different training status. Deoxygenation in the vastus lateralis increases consistently up to VO2peak in WC/E cyclists whereas it plateaus in T cyclists. This difference of muscle deoxygenation pattern could be a factor limiting performance by the trained cyclists. Results and experimental procedure may serve as a practical demonstration of how to track physiological factors limiting athletes from improving endurance in sports. This non-invasive technique could be helpful to trainers, and specific training plans could be designed to tackle these limitations. Future researches are needed to determine what type of training could improve specifically muscle deoxygenation.
The authors thank all cyclists who participated in this study.
References
The Influence of Training Status on the Physiological Responses to Exercise of Young Girls(2010). UKUniversity of Exeter. McNarry, M. A. (2010). The Influence of Training Status on the Physiological Responses to Exercise of Young Girls. (Unpublished doctoral dissertation). University of Exeter, UK. , McNarryM. A., (Unpublished doctoral dissertation).