Effects of interval training on blood pressure and endothelial function in hypertensive patients
Article information
Abstract
Background
Several studies have recently shown that high-intensity interval training (HIIT) is superior to moderate-intensity continuous exercise (MICE) for improvements on endothelial in patients with cardiovascular disease. However, little is known about its effects on changes in blood pressure, especially in hypertensive patients.
Purpose
To compare the effects of HIIT and MICE on changes in blood pressure reduction and endothelial function in hypertensive patients.
Methods
Fourteen hypertensive patients, aged 52.1±7.6, participated in this study. They tapered off their medications, if necessary, and were randomized to either HIIT (n = 7) or CME (n = 7) group. HIIT was composed of 5 sets of 3 min exercise at 80% HRR, and each interval was separated by 3 min recovery at 40% HRR. MICE was composed of 35 min exercise at 60% HRR. Both groups were designed to use same energy expenditure, and performed exercise 5 days/week for 4 weeks. Endothelial function was determined by assessing endothelial progenitor cells (EPCs) using flow cytometry and flow mediated dilation (FMD) using ultrasonography. Blood pressure (BP) and heart rate (HR) were measured at rest by using automatic blood pressure monitor. Repeated ANOVA was performed to analyze if the changes in dependent variables after training are different between HITT and MICE group. Statistical significance was at p< .05.
Results
There was a significant interaction in resting HR, but not in BP, FMD, and EPCs between groups after training. Systolic BP was significantly decreased in both HITT group (p = .012) and MICE group (p = .048) after training. However, diastolic BP and FMD was significantly decreased (p = .021) and increased (p = .049) in only HIIT group, respectively. EPCs were not significantly changed after training in both HIIT and MICE group.
Conclusion
The results of this study indicate that there is no big difference in HIIT and MICE for BP reduction, and HIIT and MICE are both effective for BP reduction in hypertensive patients. However, further researches are needed to illuminate differential effects of HIIT and MICE on BP response and endothelial function.
Introduction
It is a well-established that a sedentary lifestyle contributes to increased risk of cardiovascular disease (CVD), especially hypertension. Indeed, hypertension is a major cardiovascular risk factor, affecting about 1 billion people worldwide, and blood pressure (BP) management is essential for prevention and treatment for CVD (Chobanian 2003, Lawes, Vander Hoorn et al. 2008). Despite the well-established benefit of BP reduction in reducing the risk of these events, the extent of BP control remains poor.
Many Studies have shown that exercise training is effective lowers BP in hypertensive patients (Hagberg, Park et al. 2000, Whelton, Chin et al. 2002, Yung, Yung et al. 2009), and an inverse relationship between physical activity level and the incidence of CVD (Cameron and Dart 1994, Tanaka, Dinenno et al. 2000, Kakiyama, Sugawara et al. 2005).
Various mechanisms could be involved in the cardiovascular protective effects of physical activity, including reduction in sympathetic neural activity, arterial stiffness and improvement in endothelial function (Guimaraes, Ciolac et al. 2010). Regular aerobic exercise has shown to restore endothelial function, increase the release of nitric oxide (NO) via increased expression of endothelial NO synthase (eNOS) (Haram, Kemi et al. 2008), and increase the number of circulating endothelial progenitor cells (EPCs), promoting endothelial integrity, in healthy individuals (Van Craenenbroeck, Vrints et al. 2008) as well as established CVD patients (Gatta, Armani et al. 2012). However, the best exercise modality for better blood pressure control and improvements in endothelial function, particularly circulating EPCs levels, is not well established in hypertensive patients.
Moderate-intensity continuous exercise training (MICE) has been widely recommended for hypertension prevention and treatment (Williams 2007). Recently some studies reported that high intensity interval training (HIIT) is superior to MICE for improving endothelial function and its markers (Tjønna, Lee et al. 2008, Ciolac, Bocchi et al. 2010), arterial stiffness (Guimaraes, Ciolac et al. 2010) in hypertensive patients and normotensive individuals at high familial risk for hypertension. Although many researchers generally agree that both continuous and interval training programs can beneficially alter many physiological parameters, there is still controversy regarding the intensity, amount, and mode of exercise to yield optimal beneficial effects on hypertension. Several meta-analyses even suggest little or no intensity-dependent effect of exercise training for the reduction of blood pressure (Ciolac 2012, Kessler, Sisson et al. 2012). Furthermore, the amount of exercise was not matched in most of studies comparing different modes of exercises for the effects on blood pressure reduction. One other point which clams our attention is that most of studies including hypertensive patients did not eliminate the influence of anti-hypertensive medication on exercise-induced blood pressure reduction. These factors might cause inconsistent results in the previous studies.
Therefore the aim of this study was to compare HIIT and MICE for the impact on endothelial function and blood pressure in hypertensive patients after tapering off anti-hypertensive medications and after adjusting for the same amount of exercise.
Methods
Participants and study design
Thirty five volunteered for this study, and 29 subjects were recruited by telephone screening interview. They had not participated in any types of regular exercise training for at least 3 months were recruited for this study. They were not smoker, alcohol abuser, and obese. They also did not have uncontrolled hypertension, kidney failure, cardiovascular disease, chronic obstructive pulmonary disease and muscular skeletal disease. If participants were taken anti-hypertensive medications, they gradually withdrew their medication for 3 weeks. Among these, 8 participants did not complete the anti-hypertensive medication tapering phase and 2 participants were excluded from this study because of abnormal ECG and BP during exercise test. Thus, 19 patients (male= 10, female= 9, aged average 51.3 years) were finally enrolled in this study. The subjects were then randomly assigned to the HIIT (n=9), MICE (n=10), and underwent 4 weeks of training programs separately. However, 5 subjects of them were unable to complete the training program: 2 of HIIT group for personal reasons, 2 of MICE group for lower attendance, 1 of MICE group for incomplete posttests. Thus, total 14 subjects were included in data analyses. Before and after the intervention, all subjects completed a series of tests including measurements of resting systolic BP (SBP) and diastolic BP (DBP), flow mediated dilation (FMD), and EPCs. Before the experimental days, all subjects kept 12-hour overnight fasting, and did not drink alcohol and exercise for past 24 hours.
The Institutional Review Board (IRB) at Pusan National University approved this study, and all participants provided written informed consent. A compliance with 80% of attendance at the exercise program set as a criterion for the completing the study.
Antihypertensive medication withdrawal
The subjects who were taken anti-hypertensive medications withdrew their drugs over 3 weeks, if they and their physicians agreed to taper off their medications. The timeline for tapering off medication was a decrease by 50% for the 1st week, 75% for the 2nd week, and 100% for the 3rd week (Hajjar, Hart et al. 2013). All subjects were introduced to measure blood pressure two times a day (morning and before sleep). During tapering period, the subjects with elevated blood pressure (SBP/DBP > 140/90 mm Hg) or developed hypertension-related symptoms were excluded, and asked to resume their antihypertensive medications, and referred back to their primary care physician.
Stress test
Graded maximal exercise treadmill test was conducted to evaluate the abnormal symptoms during exercise under the supervision of the expert. All subjects were asked to refrain from both strenuous physical activities and the consumption of any stimulants (e.g., coffee and alcohol) that might influence heart rate for 24 hours prior to the exercise test and not to eat at least 2 hours before the start of the test. They underwent exercise testing on a programmable treadmill (GE CASE T2100; GE Medical Systems, Milwaukee, WI, USA) according to the protocol by Bruce et al (Bruce, Kusumi et al. 1973).
Blood pressure
Before and after training, BPs were measured using an automatic digital BP monitor (HEM-7071, OMRON). At each visit, three consecutive measurements were obtained in sitting position with 2-min interval after at least 5 min quiet rest. The average of the three readings was adopted as the representative BP value for the visit. BP measurements were conducted between 8 am and 10 am.
Endothelial function
To assess endothelium-dependent vascular reactivity, endothelial function was measured by using brachial artery FMD as previosly descibed (Corretti, Anderson et al. 2002). Two-dimensional ultrasonography (Vivid 7, General Electric, Horten, Norway) was performed in a longitudinal plane at just above the antecubital crease, using a 10-MHz probe. The Measurements were done on the left arm after a 15 min rest in the supine position. The diameter of the brachial artery was measured at the interface between the tunica media and tunica adventitia of the anterior and posterior walls. After recording baseline images, a pneumatic cuff on the forearm was inflated to 180-200 mm (50 mm Hg higher than systolic blood pressure) Hg for 5 min. The peak diameter of the brachial artery was recorded after 60–90 sec following sudden deflation of the cuff. Percent FMD induced by reactive hyperemia was expressed as the relative change from baseline (% FMD = 100× [(diameter after hyperemia-baseline diameter) / baseline diameter]). We measured each diameter three times during two heart beats, and the mean values were used for final analysis. Each diameter was measured at the peak of the R wave of the surface electrocardiogram. A blinded-independent ultrasonographer performed all measurements
Measurements of circulating EPCs
The circulating EPCs were defined by the expression of surface markers CD34+/CD117+ and their numbers were measured by fluorescence-activated cell analysis of peripheral blood sample.
Blood samples (3 ml) were drawn into heparinized tubed, and peripheral blood mononuclear cells (PBMCs) were isolated within 1 hour by Ficoll density gradient centrifugation (Histopaque 1077, Sigma-Aldrich, St. Louis, MO, USA). PBMCs were fixed in 2% paraformaldehyde, and stored at 4℃ until analysis. PBMCs were incubated and resuspended in PBS containing 2% FBS, and washed by centrifugation. PBMCs were analyzed for the expression of surface of antigens with two-color analtsis using fluorescein isothiocyanate (FITC)-conjugated and phycoerythrin (PE)-conjugated monoclonal antibodies by flow cytometric analysis. PBMCs corresponding to 1 ml peripheral blood were incubated with 100μl of FITC-conjugated anti-CE34 monoclonal antibody (#348053; BD Biosciences Pharmingen, San Diego, CA, USA) diluted 1:100 in PBS containing 2% FBS for 20 min at 4℃. EPCs were identified through their expression of CD34 and CD117. FITC-conjugated mouse IgG1 antibody (#555909; BD Biosciences Pharmingen) serves as an isotype control. PBMCs were then incubated with PE-conjugated CD117 (#555714; BD Biosciences Pharmingen) monoclonal antibodies diluted 1:100 in PBS containing 2% FBS for 20 min 4℃. PE-conjugated mouse IgG1 antibody (#349043; BD Biosciences Pharmingen) serves as an isotype control. After washing with PBS containing 2% FBSS, PBMCs were resuspended and analyzed by flow cytometry. The 30,000 cells/sample were analyzed on a FACS vantage SE flow sorter (Becton Dickinson, San Jose, California, USA). Dead cells and debris were gated out using scattering property of the cells. The percentages of CD34+/CD117+ cells were calculated by subtracting values of isotype control samples by a two-dimensional side-scatter fluorescence dot-plot analysis of the sample after gating on the lymphocyte population. Data were analyzed by using Cell Quest Pro software (Bectom Dickinson).
Exercise training
Both HIIT and MICE groups performed an aerobic exercise program (walking/running on a treadmill) 5 days/week for 4 weeks under supervision of an exercise specialist. All subjects were instructed not to add any leisure exercise during the study period. Exercise intensity was determined by the Karvonen method [Target HR = Exercise Intensity × (HRmax – HRrest) + HRrest] and calculated to promote the same cardiovascular workload for both HIIT and MICE group. The HIIT consisted of warm-up for 5 min at 40% of HRR and 5 min at 60% of HRR with light jogging followed by 5 times of 3 min intervals at 80% of HRR (at the end of the 3 min period), with a 3 min active recovery at 40% of HRR between each interval. The MICE consisted of 5 min warm-up at 40% of HRR followed by 35 min continuous running at 60% of HRR. Each individual’s HR was monitored during all exercise sessions with a HR monitor (polar RS400sd, APAC, 90026360, USA). Subjects were excluded if they did not perform more than 80% of the exercise sessions.
Statistics
Data were analysed using SPSS version 12.0 (SPSS Inc., Chicago, IL, USA). Independent t tests were used to compare baseline characteristics between HITT and MICE group. Then, repeated analysis of variance (ANOVA) was performed to analyze if the changes in dependent variables after training are different between HITT and MICE group. Paired t tests were also performed to test changes before and after training for each group. Statistical significance was at p< .05.
Results
Subjects’ baseline characteristics were shown in Table 1. Age, weight, body mass index (BMI), resting HR, and resting SBP were similar between groups before training (Table1). However, the HITT group was significantly taller (p= .046), and had significantly higher resting DBP (p= .034) than the MICE group.
Changes in weight, BMI and resting HR after training
The results of changes in weight, BMI, and resting HR after training are shown in the Table 2. There were no significant interaction, indicating differences in the changes of weight and BMI between HITT and MICE group after training. Also, weight and BMI did not change after training in each group. However, there was a significant interaction, indicating that the change of resting HR was significantly different (p= .008) between HITT and MICE group after training. Resting HR was significantly reduced after training in HITT group (p= .016), but not in MICE group.
Changes in resting SBP and DBP after training
The results of changes in resting SBP and DBP after training are shown in the Fig. 1. There were no significant interaction, indicating differences in the changes of resting SBP and DBP between HITT and MICE group after training. However, paired t tests revealed that HITT group significantly decreased resting SBP (p= .012) and DBP (p= .021) after training, while MICE group significantly decreased only resting SBP (p= .048). Although MICE group seemed to decrease resting DBP, this change was not statistically significant.
Changes in FMD and EPCs after training
The results of changes in FMD and EPCs after training are shown in the Fig. 2 and Fig. 3, respectively. . There were no significant interaction, indicating differences in the changes of FMD and EPCs between HITT and MICE group after training. However, paired t tests showed that FMD was significantly improved after training in HIIT group (p = .049), but not in MICE group (Fig. 2). EPCs seemed to be increased after training in both HIIT and MICE, but these improvements did not reach to the statistical significance (Fig. 3).
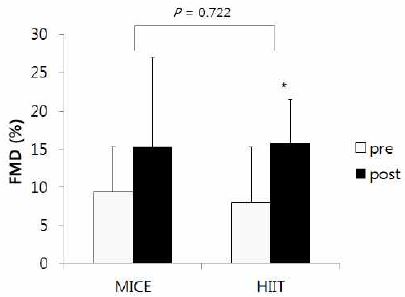
Results of changes in FMD after training
Note: FMD; flow mediated dilation, MICE; moderate-intensity continuous exercise, HIIT; high-intensity interval training
*significant difference between pre and post at p< .05
Discussion
Heart rate
Elevated resting HR is associated with an increased risk of cardiovascular disease and mortality (Palatini and Julius 2004). Additionally, individuals with an elevated resting HR are generally less fit as assessed by cardiorespiratory fitness. In this study, there was a strong interaction between the exercise mode (HITT and MICE) and 4 weeks of training on resting HR. This means that the aspect of changes in resting HR by training was different between HITT and MICE group. Indeed, the HIIT group significantly decreased resting HR after training, but MICE did not change. This result is in line with previous studies that showed greater effects of HIIT compared to MICE in improvements on cardiorespiratory fitness in healthy adults (Helgerud, Hoydal et al. 2007) as well as patients with metabolic syndrome (Tjønna, Lee et al. 2008), heart failure (Wisløff, Støylen et al. 2007), and coronary artery disease (Rognmo, Hetland et al. 2004). The superiority of HIIT for reducing resting HR has an important clinical implication because there is strong association between low cardiorespiratory fitness and incidence of hypertension (Blair, Goodyear et al. 1984, Barlow, LaMonte et al. 2006, Chase, Sui et al. 2009). Taken togather, greater decrease in resting HR in HIIT compared to MICE may indicate that HIIT could be more effective than MICE in improving cardiorespiratory fitness for the prevention and treatment of CVD including hypertension, although we did not measure other indexes of cardiorespiratory fitness such as amximal oxygene consumption.
Blood pressure
It is well known that aerobic exercise decreases blood pressure and typical MICE exerts significant reduction of BP in hypertensive adults (Williams 2007). Many studies have shown the antihypertensive effects of endurance training to be in the range of 3-10.5 mmHg for systolic BP and 2-7.6 mmHg for diastolic BP (Engström, Hedblad et al. 1999, Hagberg, Park et al. 2000, Ishikawa-Takata, Ohta et al. 2003, Yung, Yung et al. 2009). However, a few studies have analyzed the effect of different mode, particularly induced by different intensity, of exercise training on BP change. Most of studies have shown that exercise intensity generally does not influence the level of blood pressure decline (Wisløff, Støylen et al. 2007, Tjønna, Lee et al. 2008, Ciolac, Guimarães et al. 2009, Ciolac, Bocchi et al. 2010, Guimaraes, Ciolac et al. 2010). Only one study with metabolic syndrome rats demonstrated that HIIT is superior effect on BP reduction (Haram, Kemi et al. 2008). Our study is the first human study reporting that HIIT influences the magnitude of BP reduction, particularly in hypertensive patients. More importantly, these effects are independent of anti-hypertensive medications. In this study, there was no interaction between groups and training periods on neither SBP nor DBP change. This result indicates that there is no difference in BP reduction between HITT and MICE after training. However, we found that resting SBP were significantly decreased by training in both HIIT (10.6%) and MICE (8.9%) group, but resting DBP were significantly decreased only in HIIT group (13.9%). Thus, we can not eliminate the possibility that HITT may have a more favorable DBP response than MICE in hypertensive patients. At the same time, we also can not eliminate the possibility that a significant DBP reduction in HIIT in this study may be due to a significantly higher baseline DBP level in HIIT than MICE. Therefore, further reserches are required to find out which exercise program is better for BP reductiion in hypertensive patients.
Many factors are involved in the BP reduction mediated by exercise training. The decrease in sympathetic nervous system activity (Wiegman, Harris et al. 1981), improvements on baroreflex control (Somers, Conway et al. 1991) and an increase in endothelial function (NO production) (Maeda, Miyauchi et al. 2001) are probably involved in the anti-hypertensive effects of exercise. Furthermore, effects on angiogenesis (Sexton and Laughlin 1994), arterial remodeling (Dinenno, Tanaka et al. 2001) and arterial distensibility (Ferrier, Waddell et al. 2001) might be also related to the BP reduction after endurance exercise training. The HIIT group decreased DBP more than MICE group in this study, although HIIT group’s baseline DBP level was significantly higher than MICE. Perhaps it is because of differential effects on vasodilation caused by differential aspects of shear stress. Exercise-induced improvement in vasodilation function is mainly due to increases in NO production (Furchgott and Zawadzki 1980, Haram, Adams et al. 2006). An alternate low- and high-intensity exercise may produce different levels of shear stress on the walls of blood vessels repeatedly. As a result, this may stimulate endothelial more frequently, and consequently produce more NO production, and eventually improve endothelial function more. Although we did not measure NO production in this study, we did confirm increases in FMD after training, which in fact occurred only in HIIT group whose DBP was significantly decreased. This relationship is also supported by the results of previous studies, reporting the correlation between DBP and FMD (Thomas, Chook et al. 2004, Schjerve, Tyldum et al. 2008).
FMD
Exercise induced shear stress to the arterial wall stimulates endothelial to produce NO, which contributes to vasodilation. Thus, it is not surprising that aerobic exercise training increases endothelial function. Recently, some studies suggested that HIIT induces a greater shear stress compared with MICE because of frequent stimulation (Haram, Kemi et al. 2008). It supports the result of our study that the HIIT group significantly increased FMD, but the MICE did not. This result suggests that HIIT may have a greater improvement in FMD than MICE in hypertensive patients. However, we could not find a significant interaction between groups and training periods on FMD change, indicating that there is no difference in FMD change between HIIT and MICE after training. Therefore, further reserchese are needed to investigate if HIIT is indeed superior than MICE for improvements of endothelial function.
Several lines of evidence also support the result of our study. HIIT showed a greater improvement in FMD compared to MICE in individuals with diverse CVD risk factors (Wisløff, Støylen et al. 2007, Schjerve, Tyldum et al. 2008, Tjønna, Lee et al. 2008, Ciolac, Bocchi et al. 2010). NO availability and several factors that influence NO bioavailability such as blood glucose, insulin sensitivity, and oxidized low-density lipoprotein were also more effective in HIIT than MICE in metabolic syndrome patients (Tjønna, Lee et al. 2008). One of the potential mechanism underlying the superiority of HIIT for improving endothelial function is frequent shear stress generated by alternative low- and high-intensity exercise, which may yield further enhancement of the endothelial function via differential molecular responses of endothelium compared to the MICE (Haram, Kemi et al. 2008).
EPCs
Circulating EPCs participate in the maintenance of endothelial function and contribute to vascular repair (Asahara, Murohara et al. 1997, Hill, Zalos et al. 2003). EPCs number and function are inversely correlated with age and common CVD risk factors (Vasa, Fichtlscherer et al. 2001, Hill, Zalos et al. 2003). Reduced numbers and impaired function of circulating EPCs have been shown to be an independent predictor of morbidity and mortality in patients with CVD (Dzau, Gnecchi et al. 2005), whereas increased numbers of EPCs are inversely correlated with cardiovascular events (Werner, Kosiol et al. 2005, Cassese, Galasso et al. 2013).
Physical activity is a strong inductor of EPCs mobilization from the bone marrow, and promotes these cell to be located in the area of ischemia (Leosco, Rengo et al. 2007, Leone, Valgimigli et al. 2009, Ribeiro, Ribeiro et al. 2013). The effects of exercise on endothelial function and EPCs numbers and migratory activity in healty and CVD patients have been investigated by several studies (Adams, Lenk et al. 2004, Laufs, Werner et al. 2004, Laufs, Urhausen et al. 2005, Van Craenenbroeck, Hoymans et al. 2010, Mezzani, Grassi et al. 2013). Recent studies show that 10 weeks of swimming repairs the impairment of EPC in hypertensive rats (Fernandes et al., 2012), and also high-intensity interval training effectively enhances EPCs functionality in sedentary males (Tsai et al. 2016). In the present study, however, there were no significant changes on EPCs in both HIIT and MICE group. Although EPCs were increased about 100% in the HIIT group and 95% in the MICE group, these were not statistically significant. This discrepancy may be due to the large variation of the changes on EPCs and the small number of subjects in this study. It is also possible that 4 weeks of exercise training may be not long enough to improve EPCs in this study.
So far, several mechanisms have been proposed to explain the increase in EPCs as a result of exercise. An increased bioavailability of NO appears to be a key mechanism by which exercise training facilitates the mobilization of EPCs (Jenkins et al., 2009). Shear stress caused by increased blood flow stimulates the expression of endothelial nitric oxide synthesis (eNOS), and which leads to the expression of EPCs from bone marrow (Asahara et al., 1997). Nevertheless, there is insufficient studies have been conducted to draw the definitive conclusion of different types of exercise program to influence the mobilization of EPCs. Therefore, further studies are needed regarding these issues.
Conclusion
We could not find any significant interaction between HIIT and MICE group for changes in SBP, DBP, FMD, and EPCs after training. These results indicate that overall effects of HIIT and MICE on BP reduction and endothelial function are similar. However, we found that HIIT group significantly decreased resting HR, SBP, and DBP, and increased FMD, but MICE group significantly decreased only resting SBP. Therefore, further researches are required to illuminate differential effects of HIIT and MICE on BP response and endothelial function.
Acknowledgements
This work was done with the sports promotion fund from Korea Institute of Sport Science, Seoul Olympic Sports Promotion Foundation (KISS-15-A02002)
References
Adams, V., et al. (2004). Increase of circulating endothelial progenitor cells in patients with coronary artery disease after exercise-induced ischemia.” Arteriosclerosis, Thrombosis, and Vascularbiology, 24(4), 684-690.
Adams V.. et al 2004;Increase of circulating endothelial progenitor cells in patients with coronary artery disease after exercise-induced ischemia”. Arteriosclerosis, Thrombosis, and Vascularbiology 24(4):684–690. 10.1161/01.atv.0000124104.23702.a0.Asahara, T., et al. (1997). Isolation of putative progenitor endothelial cells for angiogenesis. Science, 275(5302), 964-966.
Asahara T.. et al 1997;Isolation of putative progenitor endothelial cells for angiogenesis. Science 275(5302):964–966. 10.1126/science.275.5302.964.Barlow, C. E., et al. (2006). Cardiorespiratory fitness is an independent predictor of hypertension incidence among initially normotensive healthy women. American Journal of Epidemiology. 163(2), 142-150.
Barlow C. E.. et al 2006;Cardiorespiratory fitness is an independent predictor of hypertension incidence among initially normotensive healthy women. American Journal of Epidemiology 163(2):142–150. 10.1093/aje/kwj019.Blair, S. N., et al. (1984). Physical fitness and incidence of hypertension in healthy normotensive men and women. JAMA, 252(4), 487-490.
Blair S. N.. et al 1984;Physical fitness and incidence of hypertension in healthy normotensive men and women. JAMA 252(4):487–490.Bruce, R. A., et al. (1973). Maximal oxygen intake and nomographic assessment of functional aerobic impairment in cardiovascular disease. American Heart Journal, 85(4), 546-562.
Bruce R. A.. et al 1973;Maximal oxygen intake and nomographic assessment of functional aerobic impairment in cardiovascular disease. American Heart Journal 85(4):546–562. 10.1016/0002-8703(73)90502-4.Cameron, J. D. and A. M. Dart (1994). Exercise training increases total systemic arterial compliance in humans. American Journal of Physiology-Heart and Circulatory Physiology, 266(2), H693-H701.
Cameron J. D., et al, Dart A. M.. 1994;Exercise training increases total systemic arterial compliance in humans. American Journal of Physiology-Heart and Circulatory Physiology 266(2):H693–H701. 10.1152/ajpheart.1994.266.2.h693.Cassese, S., Galasso, G., Sciahbasi, A., Scacciatella, P., Muçaj, A., Piccolo, R. ... Piscione, F. (2013). Antiplatelet theRapy after Genous EPC-capturing coroNary stenT implantatiOn: The ARGENTO Study: A prospective, multicenter registry. International Journal of Cardiology, 167(3), 757-761.
Cassese S., Galasso G., Sciahbasi A., Scacciatella P., Muçaj A., Piccolo R., et al, Piscione F.. 2013;Antiplatelet theRapy after Genous EPC-capturing coroNary stenT implantatiOn: The ARGENTO Study: A prospective, multicenter registry. International Journal of Cardiology 167(3):757–761.Chase, N. L., et al. (2009). The association of cardiorespiratory fitness and physical activity with incidence of hypertension in men. American Journal of Hypertension, 22(4), 417.
Chase N. L.. et al 2009;The association of cardiorespiratory fitness and physical activity with incidence of hypertension in men. American Journal of Hypertension 22(4):417. 10.1038/ajh.2009.6.Chobanian, A. V. (2003). Joint National Committee on Prevention, Detection, Evaluation, and Treatment of High Blood Pressure. National Heart, Lung, and Blood Institute; National High Blood Pressure Education Program Coordinating Committee: Seventh report of the Joint National Committee on Prevention, Detection, Evaluation, and Treatment of High Blood Pressure. Hypertension, 42, 1206-1252.
Chobanian A. V.. 2003;Joint National Committee on Prevention, Detection, Evaluation, and Treatment of High Blood Pressure. National Heart, Lung, and Blood Institute; National High Blood Pressure Education Program Coordinating Committee: Seventh report of the Joint National Committee on Prevention, Detection, Evaluation, and Treatment of High Blood Pressure. Hypertension 42:1206–1252.Ciolac, E. G. (2012). High-intensity interval training and hypertension: maximizing the benefits of exercise. Am J Cardiovasc Dis, 2(2), 102-110.
Ciolac E. G.. 2012;High-intensity interval training and hypertension: maximizing the benefits of exercise. Am J Cardiovasc Dis 2(2):102–110.Ciolac, E. G., et al. (2010). Effects of high-intensity aerobic interval training vs. moderate exercise on hemodynamic, metabolic and neuro-humoral abnormalities of young normotensive women at high familial risk for hypertension. Hypertension Research, 33(8), 836-843.
Ciolac E. G.. et al 2010;Effects of high-intensity aerobic interval training vs. moderate exercise on hemodynamic, metabolic and neuro-humoral abnormalities of young normotensive women at high familial risk for hypertension. Hypertension Research 33(8):836–843. 10.1038/hr.2010.72.Ciolac, E. G., et al. (2009). Acute effects of continuous and interval aerobic exercise on 24-h ambulatory blood pressure in long-term treated hypertensive patients. International Journal of Cardiology, 133(3), 381-387.
Ciolac E. G.. et al 2009;Acute effects of continuous and interval aerobic exercise on 24-h ambulatory blood pressure in long-term treated hypertensive patients. International Journal of Cardiology 133(3):381–387. 10.1016/j.ijcard.2008.02.005.Corretti, M. C., et al. (2002). Guidelines for the ultrasound assessment of endothelial-dependent flow-mediated vasodilation of the brachial artery: A report of the International Brachial Artery Reactivity Task Force. Journal of the American College of Cardiology, 39(2), 257-265.
Corretti M. C.. et al 2002;Guidelines for the ultrasound assessment of endothelial-dependent flow-mediated vasodilation of the brachial artery: A report of the International Brachial Artery Reactivity Task Force. Journal of the American College of Cardiology 39(2):257–265.Dinenno, F. A., et al. (2001). Regular endurance exercise induces expansive arterial remodelling in the trained limbs of healthy men. The Journal of Physiology, 534(1), 287-295.
Dinenno F. A.. et al 2001;Regular endurance exercise induces expansive arterial remodelling in the trained limbs of healthy men. The Journal of Physiology 534(1):287–295. 10.1111/j.1469-7793.2001.00287.x.Dzau, V. J., et al. (2005). Therapeutic potential of endothelial progenitor cells in cardiovascular diseases. Hypertension, 46(1), 7-18.
Dzau V. J.. et al 2005;Therapeutic potential of endothelial progenitor cells in cardiovascular diseases. Hypertension 46(1):7–18. 10.1161/01.hyp.0000168923.92885.f7.Engström, G., et al. (1999). Hypertensive men who exercise regularly have lower rate of cardiovascular mortality. Journal of Hypertension, 17(6), 737-742.
Engström G.. et al 1999;Hypertensive men who exercise regularly have lower rate of cardiovascular mortality. Journal of Hypertension 17(6):737–742.Fernandes, T., et al. (2012). Exercise training restores the endothelial progenitor cells number and function in hypertension: implications for angiogenesis. Journal of Hypertension, 30(11), 2133–2143
. Fernandes T.. et al 2012;Exercise training restores the endothelial progenitor cells number and function in hypertension: implications for angiogenesis. Journal of Hypertension 30(11):2133–2143.Ferrier, K. E., et al. (2001). Aerobic exercise training does not modify large-artery compliance in isolated systolic hypertension. Hypertension, 38(2), 222-226.
Ferrier K. E.. et al 2001;Aerobic exercise training does not modify large-artery compliance in isolated systolic hypertension. Hypertension 38(2):222–226. 10.1161/01.hyp.38.2.222.Furchgott, R. F. and J. V. Zawadzki (1980). The obligatory role of endothelial cells in the relaxation of arterial smooth muscle by acetylcholine. Nature, 288(5789), 373-376.
Furchgott R. F., et al, Zawadzki J. V.. 1980;The obligatory role of endothelial cells in the relaxation of arterial smooth muscle by acetylcholine. Nature 288(5789):373–376. 10.1038/288373a0.Gatta, L., et al. (2012). Effects of a short-term exercise training on serum factors involved in ventricular remodelling in chronic heart failure patients. International Journal of Cardiology, 155(3), 409-413.
Gatta L.. et al 2012;Effects of a short-term exercise training on serum factors involved in ventricular remodelling in chronic heart failure patients. International Journal of Cardiology 155(3):409–413. 10.1016/j.ijcard.2010.10.045.Guimaraes, G. V., et al. (2010). Effects of continuous vs. interval exercise training on blood pressure and arterial stiffness in treated hypertension. Hypertension Research, 33(6), 627-632.
Guimaraes G. V.. et al 2010;Effects of continuous vs. interval exercise training on blood pressure and arterial stiffness in treated hypertension. Hypertension Research 33(6):627–632.Hagberg, J. M., et al. (2000). The role of exercise training in the treatment of hypertension. Sports Medicine, 30(3), 193-206.
Hagberg J. M.. et al 2000;The role of exercise training in the treatment of hypertension. Sports Medicine 30(3):193–206. 10.2165/00007256-200030030-00004.Hajjar, I., et al. (2013). Safety and blood pressure trajectory of short-term withdrawal of antihypertensive medications in older adults: Experience from a clinical trial sample. Journal of the American Society of Hypertension, 7(4), 289-293.
Hajjar I.. et al 2013;Safety and blood pressure trajectory of short-term withdrawal of antihypertensive medications in older adults: Experience from a clinical trial sample. Journal of the American Society of Hypertension 7(4):289–293. 10.1016/j.jash.2013.04.001.Haram, P. M., et al. (2006). Time-course of endothelial adaptation following acute and regular exercise. European Journal of Cardiovascular Prevention & Rehabilitation, 13(4), 585-591.
Haram P. M.. et al 2006;Time-course of endothelial adaptation following acute and regular exercise. European Journal of Cardiovascular Prevention & Rehabilitation 13(4):585–591. 10.1097/01.hjr.0000198920.57685.76.Haram, P. M., et al. (2008). Aerobic interval training vs. continuous moderate exercise in the metabolic syndrome of rats artificially selected for low aerobic capacity. Cardiovascular Research, 81(4), 723-732.
Haram P. M.. et al 2008;Aerobic interval training vs. continuous moderate exercise in the metabolic syndrome of rats artificially selected for low aerobic capacity. Cardiovascular Research 81(4):723–732. 10.1093/cvr/cvn332.Helgerud, J., et al. (2007). Aerobic high-intensity intervals improve VO2max more than moderate training. Medicine and science in sports and exercise, 39(4), 665.
Helgerud J.. et al 2007;Aerobic high-intensity intervals improve VO2max more than moderate training. Medicine and science in sports and exercise 39(4):665. 10.1249/mss.0b013e3180304570.Hill, J. M., et al. (2003). Circulating endothelial progenitor cells, vascular function, and cardiovascular risk. New England Journal of Medicine, 348(7), 593-600.
Hill J. M.. et al 2003;Circulating endothelial progenitor cells, vascular function, and cardiovascular risk. New England Journal of Medicine 348(7):593–600. 10.1056/nejmoa022287.Ishikawa-Takata, K., et al. (2003). How much exercise is required to reduce blood pressure in essential hypertensives: A dose–response study. American Journal of Hypertension, 16(8), 629-633.
Ishikawa-Takata K.. et al 2003;How much exercise is required to reduce blood pressure in essential hypertensives: A dose–response study. American Journal of Hypertension 16(8):629–633. 10.1016/s0895-7061(03)00895-1.Jenkins et al. (2009). Effects of acute and chronic endurance exercise on intracellular nitric oxide in putative endothelial progenitor cells: role of NAPDH oxidase. American Journal of Physiology-Heart and Circulatory Physiology, 297(5), H1798-1805.
Jenkins . et al 2009;Effects of acute and chronic endurance exercise on intracellular nitric oxide in putative endothelial progenitor cells: role of NAPDH oxidase. American Journal of Physiology-Heart and Circulatory Physiology 297(5):H1798–1805. 10.1152/ajpheart.00347.2009.Kakiyama, T., et al. (2005). Effects of short-term endurance training on aortic distensibility in young males. Medicine and Science in Sports and Exercise, 37(2), 267-271.
Kakiyama T.. et al 2005;Effects of short-term endurance training on aortic distensibility in young males. Medicine and Science in Sports and Exercise 37(2):267–271. 10.1249/01.mss.0000152733.12578.5a.Kessler, H. S., et al. (2012). The potential for high-intensity interval training to reduce cardiometabolic disease risk. Sports Medicine, 42(6): 489-509.
Kessler H. S.. et al 2012;The potential for high-intensity interval training to reduce cardiometabolic disease risk. Sports Medicine 42(6):489–509. 10.2165/11630910-000000000-00000.Laufs, U., et al. (2005). Running exercise of different duration and intensity: Effect on endothelial progenitor cells in healthy subjects. European Journal of Cardiovascular Prevention & Rehabilitation, 12(4): 407-414.
Laufs U.. et al 2005;Running exercise of different duration and intensity: Effect on endothelial progenitor cells in healthy subjects. European Journal of Cardiovascular Prevention & Rehabilitation 12(4):407–414. 10.1097/01.hjr.0000174823.87269.2e.Laufs, U., et al. (2004). Physical training increases endothelial progenitor cells, inhibits neointima formation, and enhances angiogenesis. Circulation, 109(2): 220-226.
Laufs U.. et al 2004;Physical training increases endothelial progenitor cells, inhibits neointima formation, and enhances angiogenesis. Circulation 109(2):220–226. 10.1161/01.cir.0000109141.48980.37.Lawes, C. M., et al. (2008). Global burden of blood-pressure-related disease, 2001. The Lancet, 371(9623), 1513-1518.
Lawes C. M.. et al 2008;Global burden of blood-pressure-related disease, 2001. The Lancet 371(9623):1513–1518. 10.1016/s0140-6736(08)60655-8.Leone, A. M., et al. (2009). From bone marrow to the arterial wall: the ongoing tale of endothelial progenitor cells. European Heart Journal, 30(8), 890-899.
Leone A. M.. et al 2009;From bone marrow to the arterial wall: the ongoing tale of endothelial progenitor cells. European Heart Journal 30(8):890–899. 10.1093/eurheartj/ehp078.Leosco, D., et al. (2007). Exercise promotes angiogenesis and improves β-adrenergic receptor signalling in the post-ischaemic failing rat heart. Cardiovascular Research, 78(2), 385-394.
Leosco D.. et al 2007;Exercise promotes angiogenesis and improves β-adrenergic receptor signalling in the post-ischaemic failing rat heart. Cardiovascular Research 78(2):385–394. 10.1093/cvr/cvm109.Maeda, S., et al. (2001). Effects of exercise training of 8 weeks and detraining on plasma levels of endothelium-derived factors, endothelin-1 and nitric oxide, in healthy young humans. Life Sciences, 69(9), 1005-1016.
Maeda S.. et al 2001;Effects of exercise training of 8 weeks and detraining on plasma levels of endothelium-derived factors, endothelin-1 and nitric oxide, in healthy young humans. Life Sciences 69(9):1005–1016. 10.1016/s0024-3205(01)01192-4.Mezzani, A., et al. (2013). Speeding of pulmonary VO2 on-kinetics by light-to-moderate-intensity aerobic exercise training in chronic heart failure: Clinical and pathophysiological correlates. International Journal of Cardiology, 167(5), 2189-2195.
Mezzani A.. et al 2013;Speeding of pulmonary VO2 on-kinetics by light-to-moderate-intensity aerobic exercise training in chronic heart failure: Clinical and pathophysiological correlates. International Journal of Cardiology 167(5):2189–2195. 10.1016/j.ijcard.2012.05.124.Palatini, P. and S. Julius (2004). Elevated heart rate: A major risk factor for cardiovascular disease. Clinical and Experimental Hypertension, 26(7-8), 637-644.
Palatini P., et al, Julius S.. 2004;Elevated heart rate: A major risk factor for cardiovascular disease. Clinical and Experimental Hypertension 26(7-8):637–644. 10.1081/ceh-200031959.Ribeiro, F., et al. (2013). Effects of exercise training on endothelial progenitor cells in cardiovascular disease: A systematic review. American Journal of Physical Medicine & Rehabilitation, 92(11), 1020-1030.
Ribeiro F.. et al 2013;Effects of exercise training on endothelial progenitor cells in cardiovascular disease: A systematic review. American Journal of Physical Medicine & Rehabilitation 92(11):1020–1030.Rognmo, Ø., et al. (2004). High intensity aerobic interval exercise is superior to moderate intensity exercise for increasing aerobic capacity in patients with coronary artery disease. European Journal of Cardiovascular Prevention & Rehabilitation, 11(3), 216-222.
Rognmo Ø.. et al 2004;High intensity aerobic interval exercise is superior to moderate intensity exercise for increasing aerobic capacity in patients with coronary artery disease. European Journal of Cardiovascular Prevention & Rehabilitation 11(3):216–222. 10.1097/01.hjr.0000131677.96762.0c.Schjerve, I. E., et al. (2008). Both aerobic endurance and strength training programmes improve cardiovascular health in obese adults. Clinical Science, 115(9), 283-293.
Schjerve I. E.. et al 2008;Both aerobic endurance and strength training programmes improve cardiovascular health in obese adults. Clinical Science 115(9):283–293. 10.1042/cs20070332.Sexton, W. L. and M. H. Laughlin (1994). Influence of endurance exercise training on distribution of vascular adaptations in rat skeletal muscle. American Journal of Physiology, 266, H483-H483.
Sexton W. L., et al, Laughlin M. H.. 1994;Influence of endurance exercise training on distribution of vascular adaptations in rat skeletal muscle. American Journal of Physiology 266:H483–H483. 10.1152/ajpheart.1994.266.2.h483.Somers, V., et al. (1991). Effects of endurance training on baroreflex sensitivity and blood pressure in borderline hypertension. The Lancet, 337(8754), 1363-1368.
Somers V.. et al 1991;Effects of endurance training on baroreflex sensitivity and blood pressure in borderline hypertension. The Lancet 337(8754):1363–1368. 10.1016/0140-6736(91)93056-f.Tanaka, H., et al. (2000). Aging, habitual exercise, and dynamic arterial compliance. Circulation, 102(11), 1270-1275.
Tanaka H.. et al 2000;Aging, habitual exercise, and dynamic arterial compliance. Circulation 102(11):1270–1275. 10.1161/01.cir.102.11.1270.Thomas, G. N., et al. (2004). Deleterious impact of “high normal” glucose levels and other metabolic syndrome components on arterial endothelial function and intima-media thickness in apparently healthy Chinese subjects: the CATHAY study. Arteriosclerosis, Thrombosis, and Vascularbiology, 24(4), 739-743.
Thomas G. N.. et al 2004;Deleterious impact of “high normal” glucose levels and other metabolic syndrome components on arterial endothelial function and intima-media thickness in apparently healthy Chinese subjects: the CATHAY study. Arteriosclerosis, Thrombosis, and Vascularbiology 24(4):739–743. 10.1161/01.atv.0000118015.26978.07.Tjønna, A. E., et al. (2008). Aerobic interval training versus continuous moderate exercise as a treatment for the metabolic syndrome. Circulation, 118(4), 346-354.
Tjønna A. E.. et al 2008;Aerobic interval training versus continuous moderate exercise as a treatment for the metabolic syndrome. Circulation 118(4):346–354.Tsai, H.-H., et al. (2016). High-intensity Interval training enhances mobilization/functionality of endothelial progenitor cells and depressed shedding of vascular endothelial cells undergoing hypoxia. European Journal of Applied Physiology, 116(11-12), 2375-2388.
Tsai H.-H.. et al 2016;High-intensity Interval training enhances mobilization/functionality of endothelial progenitor cells and depressed shedding of vascular endothelial cells undergoing hypoxia. European Journal of Applied Physiology 116(11-12):2375–2388. 10.1007/s00421-016-3490-z.Van Craenenbroeck, E. M., et al. (2010). Exercise training improves function of circulating angiogenic cells in patients with chronic heart failure. Basic Research in Cardiology, 105(5), 665-676.
Van Craenenbroeck E. M.. et al 2010;Exercise training improves function of circulating angiogenic cells in patients with chronic heart failure. Basic Research in Cardiology 105(5):665–676.Van Craenenbroeck, E. M., et al. (2008). A maximal exercise bout increases the number of circulating CD34+/KDR+ endothelial progenitor cells in healthy subjects. Relation with lipid profile. Journal of Applied Physiology, 104(4), 1006-1013.
. Van Craenenbroeck E. M.. et al 2008;A maximal exercise bout increases the number of circulating CD34+/KDR+ endothelial progenitor cells in healthy subjects. Relation with lipid profile. Journal of Applied Physiology 104(4):1006–1013. 10.1152/japplphysiol.01210.2007.Vasa, M., et al. (2001). Number and migratory activity of circulating endothelial progenitor cells inversely correlate with risk factors for coronary artery disease. Circulation Research, 89(1), e1-e7.
. Vasa M.. et al 2001;Number and migratory activity of circulating endothelial progenitor cells inversely correlate with risk factors for coronary artery disease. Circulation Research 89(1):e1–e7. 10.1161/hh1301.093953.Werner, N., et al. (2005). Circulating endothelial progenitor cells and cardiovascular outcomes. New England Journal of Medicine, 353(10), 999-1007.
Werner N.. et al 2005;Circulating endothelial progenitor cells and cardiovascular outcomes. New England Journal of Medicine 353(10):999–1007.Whelton, S. P., et al. (2002). Effect of aerobic exercise on blood pressure: A meta-analysis of randomized, controlled trials. Annals of Internal Medicine, 136(7), 493-503.
Whelton S. P.. et al 2002;Effect of aerobic exercise on blood pressure: A meta-analysis of randomized, controlled trials. Annals of Internal Medicine 136(7):493–503.Wiegman, D. L., et al. (1981). Decreased vascular sensitivity to norepinephrine following exercise training. Journal of Applied Physiology, 51(2), 282-287.
Wiegman D. L.. et al 1981;Decreased vascular sensitivity to norepinephrine following exercise training. Journal of Applied Physiology 51(2):282–287. 10.1152/jappl.1981.51.2.282.Williams, L. (2007). Physical activity and public health. Circulation, 116(9), 1081-1093.
Williams L.. 2007;Physical activity and public health. Circulation 116(9):1081–1093.Wisløff, U., et al. (2007). Superior cardiovascular effect of aerobic interval training versus moderate continuous training in heart failure patients. Circulation, 115(24), 3086-3094.
Wisløff U.. et al 2007;Superior cardiovascular effect of aerobic interval training versus moderate continuous training in heart failure patients. Circulation 115(24):3086–3094.Yung, L. M., et al. (2009). Exercise, vascular wall and cardiovascular diseases. Sports Medicine, 39(1), 45-63.
Yung L. M.. et al 2009;Exercise, vascular wall and cardiovascular diseases. Sports Medicine 39(1):45–63. 10.2165/00007256-200939010-00004.